Alex Toyryla was 12 years old when he first learned that something hidden could kill him. In 2013, his family moved to Laos, a nation upon which 2.5 million tons of U.S. munitions were dropped during the Vietnam War — and about a third of those bombs failed to detonate. They are scattered throughout Laos, buried under farmland or lodged in gullies, ready to explode.
Now a senior physics major at William & Mary, Toyryla is working with a team of William & Mary physicists on a project funded by the U.S. Department of Defense’s Army Research Office with the goal of developing a device to see invisible threats, such as unexploded ordnance.
“We dropped a lot of bombs over there, and they’re still causing so many problems,” Toyryla said. “Progress on getting rid of them has been extremely slow over the last 50 years, so one potential application for what we’re developing could be to help pinpoint the location of all these munitions.”
Toyryla is careful to speak in hypothetical terms because what the team of researchers is developing has extremely broad application potential — everything from assisting in cardiac surgeries to spotting oil reserves, submarines and WMDs. They are designing a device with the capacity to see what is invisible to the naked eye.
For the most part, when people think about visibility, they think about the light they can see, explained W&M Professor of Physics Irina Novikova, who is a principal investigator on the project. For quantum physicists like Novikova, visibility represents the shorter waves within a much larger ocean of electromagnetic radiation.
Both magnetic and electric fields interact with matter, but the interaction of an electric field is usually about 100 times stronger than that of the magnetic field, which is why most modern detectors are focused on the “electro” side of optics. Novikova, her students and collaborators at the National Institute of Standards and Technology (NIST) and NASA’s Jet Propulsion Laboratory are developing a detector tuned to the “magnetic” side.
“Our project is to build an atomic magnetometer, which means a device which measures magnetic field based on atoms,” Novikova said. “What we are doing is very different from a lot of research going on in this area, because we want to measure not only the magnitude of the magnetic field with good precision, but also its direction. That provides very valuable information about whatever object you’re studying.”
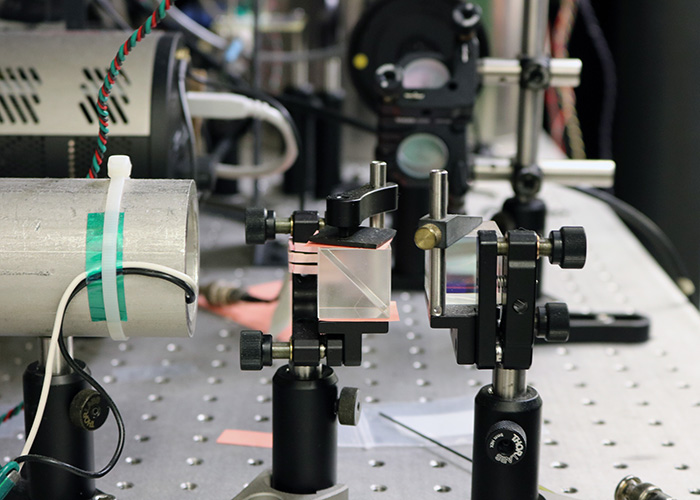
Magnitude and direction
Existing atomic magnetometers only measure the magnitude of a magnetic field, but not its direction, Novikova explained. This gives an incomplete picture of how an object interacts with a magnetic field, especially when it interacts with a large magnetic field over a long period of time.
“Every magnetic field is a vector,” explained Mario Gonzalez Maldonado, a lead on the project and a postdoc researcher in W&M’s Department of Physics. “That means you need to know both its strength and its direction. You cannot say you really know what’s going on if you are only measuring change in one of those variables.”
Gonzalez Maldonado is running experiments and analyzing data through artificial intelligence to drill down and visualize the smallest possible magnetic variation – roughly the size of the magnetic field created by a beating human heart. The smaller the change he can detect, the larger the range of hidden objects he can spot, which is essential to the project, Novikova explained, because everything on the planet interacts with Earth’s magnetic field in some way.
“Right now, existing atomic magnetometers and some superconducting magnetometers are designed for measuring very small magnetic fields, which means that when it comes to measuring changes in Earth’s magnetic field, there is a problem,” Novikova said. “Our method is intrinsically compatible in operating in Earth’s magnetic field and looking at the small changes compared to the Earth’s magnetic field.”
Novikova is quick to add that she and her fellow physicists are still in the exploratory stage of the project, which is in the first year of an expected four-year timeframe. She likens the work to designing a tuning fork, which requires constant discovery and reinterpretation as the team learns more about the frequencies it can transmit and receive.
“In a way, this is a kind of resonance tuning, but for matter not sound,” she explained. “Small signals at the right frequency give a large optical response. Hearing is a good analogy, though, because we triangulate to make sense of noise. We listen for changes in frequency or pitch and we also listen for changes in amplitude or magnitude, if it’s getting louder. We can’t have a clear picture of what we are hearing unless we account for multiple variables at once.”
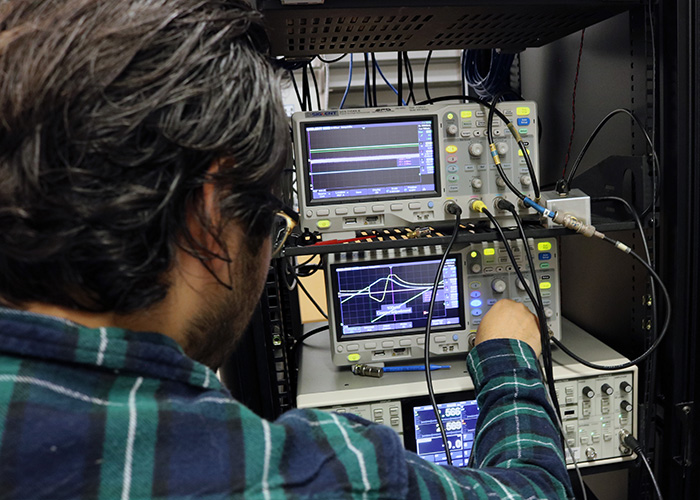
Detection and navigation
For the Department of Defense, the detection of magnetic anomalies to help locate hidden objects like submarines or centrifuges is clearly one promising potential application for the device, Novikova said, but the device could also work in reverse. For example, if a variable such as the local magnitude of the Earth’s magnetic field is known, then the device could be programmed to solve for direction.
“This would mean the device could be a powerful navigation tool,” Novikova explained. “It would use the Earth’s field instead of GPS, which is also obviously something that also appeals to the DoD — GPS-less navigation.”
The precise measurement of magnetic fields requires the use of a variety of instruments, all taking advantage of a broad range of physical phenomena, Novikova explained. The team fires infrared lasers, set to specific frequencies, through a series of lenses into a crockpot-sized magnetic shielding chamber containing rubidium atomic vapor. The vapor interacts with the laser light in such a way that, based on how it is absorbed, it can be used to determine magnetic field strength and direction.
“When we change the angle of the magnetic field, or the direction of it, then the transmission through the chamber changes at specific frequencies, so that gives us the basis for the angle and strength of the field compared to our system at any given time,” said Toyryla, who will be joining the optics group at Johns Hopkins Applied Physics Laboratory after he graduates this month.
The ultimate goal of the magnetometer device is to combine accuracy, long-term stability and vector measurement capability into one single sensing unit roughly the size of a computer chip, Toyryla explained. The device will be electromagnetically silent and produce no fields whatsoever outside the sensor reading. At such a small scale, it will both be commercially viable and have a small enough system to limit interference.
“We use atomic physics to build sensors because atoms are really reliable,” Novikova said. “An atom is an absolute tuning fork, which doesn’t change its tone and we know quite well which frequencies it can ring. They are very stable and isolated from the environment, so temperature and pressure don’t affect them. If you want to measure something with extreme accuracy, you link to atomic frequencies. What we’re developing is going to be a very precise device.”
Adrienne Berard, Assistant Director for Research, News & Analytics